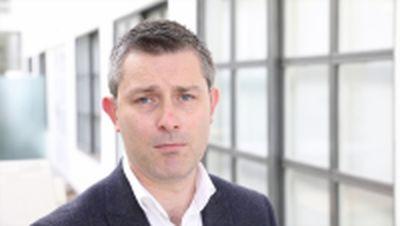
Mark Cunningham is the recently appointed Ellen Mayston Bates Professor of Neurophysiology of Epilepsy at Trinity College Dublin. In this piece, he explains the importance of neuroscience in treating epilepsy. ‘
Electricity is life but electricity is an invisible fist punching up your spine, knocking your brains right out of your skull.’
Ray Robinson
The quote above captures the main theme from Ray Robinson’s debut novel ‘Electricity’ – an evocative and unsentimental description of living with epilepsy. Through the narrator and protagonist, Lily O’Connor, the reader is given an insight into Lily’s attempts to deal with her difficult to control seizures as they impact on her life. Lily’s complex wider existence is soothed by a romantic liaison with an electrician – a girl with abnormal electrical discharges rescued by an electrician?
Can studying the abnormal electrical discharges associated with epilepsy provide insights that might provide better treatments for those living with epilepsy? This question is central to the research that my laboratory at Trinity College Dublin hopes to address. Despite there being more than 30 different types of seizures, all share the fact that underlying the seizure is a ‘misfiring’ of brain cells. This is due to alterations in how these cells generate electrical activity and communicate between each other. These electrical and chemical disturbances give rise to a disorganisation of the electrical signals between large numbers of brain cells producing an electrical storm in the brain. Such uncontrolled electrical storms cause a change in awareness, movement or sensation – a seizure.
The brain is a complex organ with 100 billion brain cells and 0.15 quadrillion connections, or synapses, between those brain cells. Remarkably, the circuits of brain cells which produce a seizure in the whole brain can be studied at a much more basic level. For example, brain cells, or neurons, can be grown in a petri dish and are capable of forming connections between themselves that are similar to those connections in the intact brain. This cell model can be used to study seizure activity in the lab and is an important tool to assess potential anticonvulsant features of new drugs. Similarly, thin slices (0.5-1 mm) of brain tissue can be prepared and kept alive in such a way that the electrical activity of seizures generated by these intact brain tissue sections can be examined. The type of seizure activity seen in these slices relates directly to seizure observed in the intact brain and we can also study this using animal models of epilepsy. A variety of models of epilepsy are available for scientists to study. These range from models in which chemical agents, termed proconvulsants, are administered to the animal which then goes on to exhibit spontaneous seizures. Other models include where genetic manipulations associated with epilepsy have been made. We also work with models of brain tumours or traumatic brain injury, both conditions in which epilepsy is commonly seen.
How do we study epilepsy? As mentioned above, seizures arise from alterations in how brain cells generate electrical activity and communicate as networks. In order to eavesdrop on this electrical activity we use an approach called neurophysiology. Neurophysiology is the study of the functions of the nervous system. This technique is directed towards unravelling the question of operation of individual neurons, connections between neurons, interactions between groups of neuron and the emergent network features that arise from all these levels of function. All of the afore mentioned levels give rise to patterns of electrical activity which can be recorded by electrodes placed on the scalp – commonly known as the electroencephalogram or EEG. The activity we study in the laboratory is therefore directly related to this clinical measurement.
Using these approaches my group aims to carry out research that addresses fundamental questions about epilepsy but also has the capacity to translate findings into the clinic and ultimately improve the treatment of people with epilepsy.
An example of this type of work is as follows. Within the brain there is a molecule which acts to help neurons communicate with each other. The molecule, glutamate, is a neurotransmitter and when released from the endings of one neuron it acts on another neuron to excite it. This excitation causes neurons to generate electrical signals or spikes and too much glutamate can cause uncontrollable electrical spiking. If unchecked these spiking neurons can produce the electrical storms that underlie seizure generation. Normally, levels of glutamate are tightly regulated in the brain however in certain conditions glutamate levels can become very high. This frequently occurs around brain tumours particularly in the case of low grade gliomas. Patients with this form of brain tumour experience epileptic seizures and it is thought that their seizures are due to an excess of glutamate. We work with slices of live human brain tissue obtained during an operation to remove this type of brain tumour and we have been using neurophysiology to record seizures in these human brain slices and study the role that glutamate is playing. Our work suggests that currently available drugs, which target a particular type of glutamate receptor, may be better at limiting seizures associated with brain tumours than other drugs presently used.
As captured in the quote at the start of this article electricity plays a fundamental role in epilepsy. I aim to carry out research which shines a light on the abnormal electrical discharges which give rise to the epilepsy. In doing so I hope to provide answers about how epilepsy occurs but more importantly use this knowledge to improve how the condition can be treated for the benefit and wellbeing of patients, their carers and families.